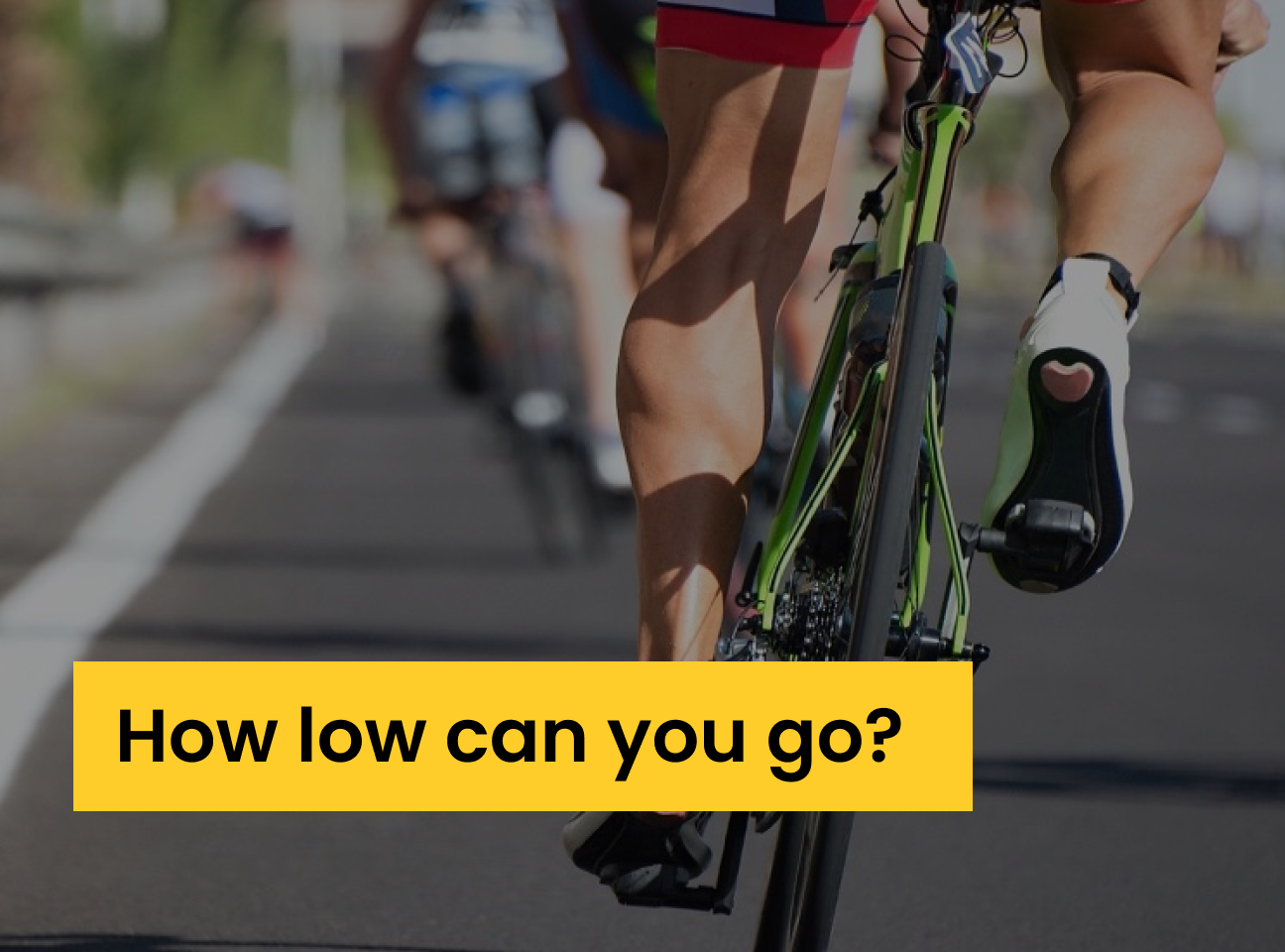
How low can you go?
Male endurance athletes are more resilient to lower levels of energy availability compared to females.
By Alex Ritson MSc, Head of Education
To read the full paper, click here: Reducing energy availability in male endurance athletes: a randomised trial with a three-step energy reduction by Jurov, Keay & Rauter (2022) (open access)
Introduction
You may call yourself a ‘sports nutritionist’ and describe your clients as ‘elite athletes’, but these classifications can invariably be disputed depending on regulations and definitions. However, one thing is for sure, we are all homo sapiens — a fact that stretches ~300,000 years deep within our DNA. As homo sapiens and previous genuses before us, we’ve spent most of our evolutionary existence as hunter-gatherers, giving our DNA ample time to develop internal mechanisms to combat spells of food shortage. This is achieved by our brain tactfully partitioning stored Calories towards survival-critical body systems — think cardiac output and nervous system activity — when the body recognises a food shortage while decreasing the stored Calories provided to non-essential survival systems — think reproduction and muscle-building processes (Wade & Jones, 2004) (see Figure 1). These genetic modifications have allowed our ancestors to survive, reproduce, and pass down their genes to us. Elite athletes are the “cream of the crop” in terms of gene pools, yet ironically, the genetic adaptations that combat spells of low energy availability (EA) impede their health and performance.
In sport and exercise science (SES), EA refers to the total oxidisable energy (Calories) remaining to support physiological processes once the difference between dietary energy intake minus exercise energy expenditure has been equated (Loucks et al., 2011). Female and male athletes (recreational and elite) competing in sports that emphasise leanness (e.g., combat sports) or with a high metabolic cost (e.g., endurance sports) — whether deliberately, compulsively, or unintentionally — commonly demonstrate signs of low EA (Dipla et al., 2020).
Figure 1. Partitioning of metabolic fuels according to priority. 1) Some processes are essential for survival and cannot be compromised. 2) Others can be reduced to achieve energy savings. 3) Reproduction and fat storage can be maladaptive when calories are scarce and have a low priority for energy distribution. Adapted from Wade & Jones (2004)
Research on low EA in SES initially focused on exercising girls and women who showed signs of poor bone health and reproductive suppression, which gave rise to the ‘Female Athlete Triad’ (Triad) (Yeager et al., 1993). As shown in Figure 2a, the Triad has evolved to fall on a bi-directional continuum from health to disease, with research demonstrating a clinical interrelatedness between symptoms (de Souza et al., 2014). In 2014, evidenced-informed guidance on the clinical management and treatment of the Triad was put forth by the Female and Male Athlete Triad Coalition for females (de Souza et al., 2014). In the same year, another concept referred to as ‘Relative Energy Deficiency in Sport’ (RED-S) was proposed (See Figure 2b), unifying a broader interconnected spectrum of more health and performance decrements associated with low EA in women and male athletes (Mountjoy et al., 2014). At present, the most clinically significant, evidence-based effects of low EA remain within the Triad in female athletes, with emerging evidence in men (Burke et al., 2018; de Souza, Koltun, & Williams, 2019; Fredericson et al., 2021; Lundy et al., 2022; Nattiv et al., 2021; Tenforde et al., 2016). Though data on low EA in male athletes remains limited, the same issues that cause the Triad and symptoms of RED-S in female athletes also apply to males. These include disordered eating, exercise dependence, and involvement in sports centred on weight-making (e.g., boxing and martial arts), aesthetics (e.g., bodybuilding), and endurance (e.g., cycling and long-distance running).
Figure 2a. The bi-directional relationship of the Triad in female athletes. Adapted from de Souza et al. (2014). Figure 2b. Health Consequences of Relative Energy Deficiency in Sport (RED-S) showing an expanded concept of the Female Athlete Triad. Adapted from Mountjoy et al. (2014)
Calculating EA requires the collation of an athlete’s dietary energy intake (kCal) and deducting these values from exercise energy expenditure (kCal) and then for these outcomes to be expressed relative to a measure of fat-free mass (FFM; kCal/ kg FFM/d) — ‘FFM’ due to its greater capacity for energy expenditure compared to fat mass. Short-term (4 – 5 days) controlled laboratory conditions have previously determined a threshold of low EA as 30 kCal’s/kg LBM in healthy, regularly menstruating, nonobese, sedentary women (Loucks & Thuma, 2003). This series of laboratory studies led by Dr Anne Loucks from Ohio University provided seminal evidence of the significance of EA in women and determined 30 kCal’s/kg LBM as the threshold by which pulsatile rhythm of luteinising hormone (LH – a proxy for gonadotropin-releasing hormone neuronal activity) becomes impaired, in addition to severe endocrine and metabolic alterations (Ihle & Loucks, 2004) (see Figure 3). The preclusion of 30 kCal/kg FFM as a threshold for reproductive dysfunction has remained prominent in the framework of the Triad and RED-S. Although the 30 kCal/kg FFM index has been shown to discriminate severe ovarian suppression from eumenorrheic females in exercising women (Reed et al., 2015) and predict more than 50% of exercising individuals with varying degrees of menstrual disturbance (Lieberman et al., 2018), a universal threshold of low EA at 30 kCal’s/kg FFM in exercising women isn’t supported by the literature outside laboratory conditions (de Souza, Koltun, Strock, et al., 2019). Instead, an EA index of 30 kCal’s/kg FFM has been suggested as a proxy to indicate an increased likelihood of energy deficiency in large groups of females (De Souza, Koltun and Williams, 2019) or as a preliminary indicator of an ‘at-risk’ profile. The current opinion is that low EA falls within a range for each individual, with sufficient EA on one side of the range supporting health and performance and insufficient EA on the other side increasing the chance of physiological and performance impairments (de Souza, Koltun, Strock, et al., 2019). This can also be viewed as a dose-response continuum, whereby as EA decreases, the probability of clinical outcomes of the Triad and RED-S increases.
Figure 3. A dose-response relationship between EA and body functions in healthy females.
Current evidence suggests that a lower EA is required to cause reproductive dysfunction and impaired bone metabolism in males compared to females (Koehler et al., 2016; Papageorgiou et al., 2017); however, the evidence remains sparse in terms of what a definitive level of EA could be to cause clinical outcomes of RED-S and the Triad. Some researchers suggest this level could be between 15 and 30 kCal/kg.FFM.d-1, however, this remains speculative. Therefore, this study aimed to determine a threshold of EA in male athletes by subjecting well-trained and elite male endurance athletes to three different magnitudes of EA. While the researchers of this study could not perform 24hr determinants of LH pulsatility as per the seminal work by Loucks et al, they did use other biomarkers of energy status established as primary and secondary clinical markers of low EA in male athletes (Lundy et al., 2022). Let’s take a look:
Methods
This was a repeated measure, randomised study design. Twelve male (18-35 years) well-trained and elite cyclists and triathletes (V O2 max between 55-64.9 mL/kg/min) with stable weight and no pre-existing dietary regimen participated in the study. Table 1 displays the study’s primary, secondary, tertiary, and quaternary outcomes.
Table 1. Measured outcomes of the study
Referenced in table:
Participants were randomly assigned to one of three low EA stages (EA reduced by 25%, 50%, or 75% from baseline for 14 days), with a one-month wash-out period between each stage to ensure the athlete’s results recovered to baseline values. At stage 0, the participant’s dietary intake was monitored using a weighed/image-based food diary, and their exercise expenditure (~1172 kCal/day) was measured using a Polar heart rate monitor. During stage 0, the researchers tracked participant outcomes at baseline to ensure each athlete met the inclusion requirements, such as minimal body weight variations. Following 14-days of baseline measurements, participants were randomly assigned to one of three low EA conditions, with each athlete’s energy intake remaining unchanged from baseline but with a prescribed duration and intensity of running or cycling daily to impose the EA deficit (25%, 50% or 75% below baseline values). An overview of the measurement outcomes and timeline of a stage of the intervention is shown below:
Figure 4. Stages 1-3 (14-day timeline).
Results
To recap, the research aimed to determine an EA threshold that increases the likelihood of health and performance impairments in male endurance athletes. With substantial heterogeneity between participants, there was no definite low EA threshold; as indicated in Table 2, perturbations in outcomes existed on a sliding scale. Well-being, explosive power output and lactate metabolism reduced from 25% of baseline EA, with trends for reductions in haemoglobin, iron, and IGF-1. At 50% from baseline EA, impairments in eating behaviour and decreases in body fat were added to the list of outcomes. At 75% from baseline EA, T3 and testosterone levels reduced and cognitive restriction increased. Reducing EA in the three levels did not elicit a significant change in mREE/pREE, which is contrary to previous studies in exercising women with signs of energy deficiency (reproductive dysfunction) (see Box 3) (Strock et al., 2020). Notably, the 75% reduction from baseline EA only lasted ten days due to certain athletes being unable to finish the intervention period, with “hunger”, “empty energy reserves”, and “inability to train at the same intensity levels as expected” being among the reasons stated by athletes. As a result of the shorter period in stage 3, not many blood parameters reached statistical significance. Interestingly, no changes were detected in reports of functional measures of testosterone in athletes (e.g., morning erections — as shown in the well-being questionnaire), which has previously been shown to be a symptom of low testosterone in exercising males (Dwyer et al., 2019). However, the researchers suggest that the discrepancy could be due to athletes’ unwillingness to share private information.
Table 2. Summary of the main findings in three-stage EA reduction phases.
Recommended reading:
Indices of Resting Metabolic Rate Accurately Reflect Energy Deficiency in Exercising Women by Strock et al. (2020) (open access)
Summary
The main objective of this study was to discern whether male athletes’ low EA is aligned with a specific numerical threshold, akin to the initial laboratory experiments in exercising women at Ohio University. As per recent evidence in exercising women, when assessed outside of laboratory conditions, low EA in male athletes occurs within a range (2-32 kCal/kg.FFM.d-1) rather than a precise threshold. Per previous findings, physiological and performance decrements appear significantly lower on the EA scale than in women. Another notable finding from the study was the potential utility of well-being and eating behaviour questionnaires for discerning early signs of low EA in male athletes, which could be used with blood markers of energy status such as IGF-1, T3, and testosterone. Conversely, the metabolic state of athletes using the mREE/pREE ratio did not trend with low EA stages. With that said, the authors state that the lack of an association between mREE/pREE could be due to the close timeframe (~12 hrs) from the athlete’s last training session, with elevations in metabolism occurring due to excess-post oxygen consumption potentially confounding the measurement. Another noteworthy observation is that the mean EA for athletes at stage 0 was ~27 kCal/kg.FFM.d-1, with athletes displaying no signs of low EA per the inclusion criteria requirements. This observation agrees with the consensus that the level of EA required for optimal performance and health is likely lower in men (Melin et al., 2019).
The study is limited by its small sample size, as shown in the Paper-2-Podium grading rubric, confounding the ability to generalise the findings and the capacity to identify relationships between energy status markers and the intervention. Furthermore, a 10-to-14-day intervention limits the scope of the results, as some athletes undergo periods of low EA for longer timeframes. It’s worth noting that the participant’s exercise energy expenditure was 1172 kCal/day (stage 0), 1465 kCal/day (stage 1), 1758 kCal/day (stage 2), and 2051 kCal/day (stage 3), which limits the findings translational potential to most athletes, given that most athletes’ daily exercise expenditure falls far below these values. With that aside, the key take homes from this research are that male athletes are more resilient to lower levels of EA, and low EA appears to appear within the range of 2-32 kCal/kg.FFM.d-1 — although the researchers suggest a narrower range (9-25 kCal/kg.FFM.d-1) in practice, considering low EA usually occurs for longer periods. Moreover, as EA reduces, changes in psychology and performance (well-being, eating behaviour, and power output) were the initial signs of low EA (25% below baseline EA), with alterations in physiological parameters (IGF-1, T3, and testosterone) occurring as EA reduced further (50-75% below baseline EA).
Paper-to-Podium Matrix Analysis:
Next steps:
The study was limited by its small sample size and the duration of each intervention period; thus, studies that address these two constraints will provide more insight and practically useful knowledge. Furthermore, future studies should investigate the impact of moderate deficits in EA (25-40%) and imposing the deficit using a combination of dietary restriction and exercise energy expenditure (typical methods for imposing an energy deficit) rather than exercise alone.
Science to practice:
The findings of this paper offer nutrition practitioners strategies for prescribing EA targets during an energy deficit and monitoring EA outcomes throughout an intervention. Consider a male endurance athlete who needs a nutritional intervention to lose body fat for performance reasons. Based on this research, the initial objective before the intervention should be to undertake an EA screening using a well-being questionnaire, body composition measurements, and blood markers related to energy status (such as IGF-1, T3, and testosterone). If the athlete’s EA profile shows no signs of low EA based on the measured outcomes, the practitioner can then assign the athlete an energy deficit, aiming to keep the athlete’s EA deficit <50% from baseline. EA deficits >50% should be avoided, even for short periods (e.g., ten days), to avoid impaired endurance performance and physiological function. Furthermore, the athlete’s EA status should be routinely evaluated by blood measures, with reductions beyond measurement error (even if they still fall within the reference range) potentially indicating low EA. Notably, when exercise performance is a priority, the athlete’s EA should be returned to baseline, as even a 25% deficit in EA was shown to cause impairments in power output. Figure 5 provides a hypothetical overview of translating these findings into practice.
Figure 5. A hypothetical overview of a weight-loss intervention in a triathlete
The above scenario depicts a triathlete before, during, and after modifying their body composition by 3 kgs to improve their running and cycling efficiency. The athlete’s baseline EA was 36 kCal/kg. FFM.d-1. Baseline assessments of body composition (via DXA and skinfold measures), blood hormones (IGF-1, T3, total testosterone, cortisol), and a subjective measure of well-being via a questionnaire were performed. These measurements indicated that the athlete was in an adequate EA range for their health and performance, allowing a nutritional intervention to reduce the athlete’s body mass to proceed. The objective was to decrease the athlete’s body mass over eight weeks by 3 kgs, resulting in a rate of weight loss of 0.6% p/wk — a rate of weight loss previously shown to be beneficial in terms of lean body mass and performance maintenance (Garthe et al., 2011). The athlete’s EA was reduced by -23% over the first 6 – 7 weeks, with a further -5% (-28%) reduction from baseline during the final week of the nutritional intervention. Notably, the athlete’s EA never fell below -30% from baseline in an attempt to prevent any significant changes in the athlete’s blood hormonal levels. Midway through the intervention, energy status was determined using body mass, Σ8 skin fold (ISAK), IGF-1, T3, total testosterone, cortisol, and well-being via questionnaire, and at the end of the intervention using body mass and the well-being questionnaire. The athlete’s EA was reassessed at the end of the intervention using measures of body mass, Σ8 skin fold (ISAK), IGF-1, T3, total testosterone, cortisol, and the well-being questionnaire. After reaching the body composition target for over three days, the athlete’s EA was restored to baseline levels (36 kCal/kg. FFM.d-1) after adjusting for changes in body mass, lean body mass, and subsequent exercise energy expenditure.
FAQ:
Is there a validated questionnaire for screening male athletes for low EA?
It’s been long recognised that determining an athlete’s EA via the assessment of dietary energy intake, exercise energy expenditure and fat-free mass is fraught with potential errors, not to mention time-consuming. Therefore, researchers and practitioners alike have gravitated towards surrogate markers of energy status such as metabolic function, bone health, current body fat, blood markers, and questionnaires on wellbeing. While clinical indicators of low EA in male athletes have been established (see Table 3), no questionnaires have been validated for identifying male athletes with low EA. With that said, questionnaires have been proposed, such as the Male Athlete Triad cumulative risk assessment (CRA) tool for identifying bone health and eating disorder risk, the ADAM-Q for identifying reproductive dysfunction, the Sport-Specific Energy Availability Questionnaire Interview (SEAQ-I) for male cyclists, and the RED-S Specific Screening Tool (RST); however, none have been validated for identifying low EA in male athletes. To address this issue, centres in Australia, Norway, Denmark, and Sweden collaborated to develop and validate a screening tool called the Low Energy Availability among Males Questionnaire (LEAM-Q) in sub-elite and elite male athletes. The questionnaire contained questions pertaining to symptoms of low EA, such as dizziness, thermoregulation, gastrointestinal symptoms, injury, illness, well-being, recovery, sleep, and sex drive. However, following its trial in a total of 405 male athletes, it failed to clearly distinguish between athletes considered to have low EA and controls. Notably, the only sub-component within the questionnaire that could identify male athletes with low EA were questions pertaining to sex drive. With this in mind, evidence suggests that in accordance with routinely measuring some markers of energy status, as shown in Figure 5, practitioners may also want to consider monitoring athlete’s subjective assessments of their sex drive (see Table 4 for the questions used in the LEAM-Q questionnaire).
Table 3. Definition of Clinical Indicators of LEA.
Retrieved from Lundy et al. 2022.
Table 4.
Retrieved from the LEAM-Q questionnaire by Lundy et al. (2022).
Take home:
A range exists for low EA in endurance males, which is lower than the proposed threshold in women. According to this research, this range is most likely between 9 and 25 kCal/kg FFM.d-1 in endurance athletes, which practitioners can use as an indicator of potential low EA status when working with endurance athletes. Furthermore, when athletes incur low EA, perceptions of well-being and drops in performance may be the first indirect indicators of low EA, followed by changes in eating behaviour, body composition and blood hormones. Finally, indirect measures of energy status may be effective for measuring low EA in endurance athletes, such as the well-being questionnaire, body composition measurements (e.g., reduced body mass), and blood markers related to energy status, such as IGF-1, T3, and testosterone.
References:
Burke, L. M., Close, G. L., Lundy, B., Mooses, M., Morton, J. P., & Tenforde, A. S. (2018). Relative energy deficiency in sport in male athletes: A commentary on its presentation among selected groups of male athletes. International Journal of Sport Nutrition and Exercise Metabolism, 28(4), 364–374. https://doi.org/10.1123/ijsnem.2018-0182
Burke, L. M., Lundy, B., Fahrenholtz, I. L., & Melin, A. K. (2018). Pitfalls of conducting and interpreting estimates of energy availability in free-living athletes. International Journal of Sport Nutrition and Exercise Metabolism, 28(4), 350–363. https://doi.org/10.1123/ijsnem.2018-0142
de Souza, M. J., Koltun, K. J., Strock, N. C., & Williams, N. I. (2019). Rethinking the concept of an energy availability threshold and its role in the Female Athlete Triad. Current Opinion in Physiology, 10(April), 35–42. https://doi.org/10.1016/j.cophys.2019.04.001
de Souza, M. J., Koltun, K. J., & Williams, N. I. (2019). The Role of Energy Availability in Reproductive Function in the Female Athlete Triad and Extension of its Effects to Men: An Initial Working Model of a Similar Syndrome in Male Athletes. Sports Medicine, 49(s2), 125–137. https://doi.org/10.1007/s40279-019-01217-3
de Souza, M. J., Lee, D. K., VanHeest, J. L., Scheid, J. L., West, S. L., & Williams, N. I. (2007). Severity of energy-related menstrual disturbances increases in proportion to indices of energy conservation in exercising women. Fertility and Sterility, 88(4), 971–975. https://doi.org/10.1016/j.fertnstert.2006.11.171
de Souza, M. J., Nattiv, A., Elizabeth, J., Misra, M., Williams, N. I., Mallinson, R. J., Gibbs, J. C., Olmsted, M., Goolsby, M., Matheson, G., Barrack, M., Burke, L., Drinkwater, B., Lebrun, C., Loucks, A. B., Mountjoy, M., Nichols, J., & Borgen, J. S. (2014). 2014 Female Athlete Triad Coalition Consensus Statement on Treatment and Return to Play of the Female Athlete Triad. Current Sports Medicine Reports, 13(4), 219–232. https://doi.org/10.1249/JSR.0000000000000077
Dwyer, A. A., Chavan, N. R., Lewkowitz-Shpuntoff, H., Plummer, L., Hayes, F. J., Seminara, S. B., Crowley, W. F., Pitteloud, N., & Balasubramanian, R. (2019). Functional hypogonadotropic hypogonadism in men: Underlying neuroendocrine mechanisms and natural history. The Journal of Clinical Endocrinology & Metabolism, 104(August), 3403–3414. https://doi.org/10.1210/jc.2018-02697
Fredericson, M., Kussman, A., Misra, M., Barrack, M. T., de Souza, M. J., Kraus, E., Koltun, K. J., Williams, N. I., Joy, E., & Nattiv, A. (2021). The Male Athlete Triad—A Consensus Statement From the Female and Male Athlete Triad Coalition Part II. Clinical Journal of Sport Medicine, Publish Ah(June). https://doi.org/10.1097/jsm.0000000000000948
Garthe, I., Raastad, T., Refsnes, P. E., Koivisto, A., & Sundgot-Borgen, J. (2011). Effect of Two Different Weight-Loss Rates on Body Composition and Strength and Power-Related Performance in Elite Athletes. International Journal of Sport Nutrition and Exercise Metabolism, 21(2), 97–104. https://doi.org/10.1123/ijsnem.21.2.97
Hooper, S. L., & Mackinnon, L. T. (1995). Monitoring Overtraining in Athletes Recommendations. In Sports Med (Vol. 20, Issue 5).
Ihle, R., & Loucks, A. B. (2004). Dose-response relationships between energy availability and bone turnover in young exercising women. Journal of Bone and Mineral Research, 19(8), 1231–1240. https://doi.org/10.1359/JBMR.040410
Koehler, K., Hoerner, N. R., Zinner, C., de Souza, M. J., Schaenzer, W., Braun, H., & Gibbs, J. C. (2016). Low energy availability in exercising men is associated with reduced leptin and insulin but not with changes in other metabolic hormones. Journal of Sports Sciences, 34(20), 1921–1929. https://doi.org/10.1080/02640414.2016.1142109
Lieberman, J. L., de Souza, M. J., Wagstaff, D. A., & Williams, N. I. (2018). Menstrual Disruption with Exercise Is Not Linked to an Energy Availability Threshold. Medicine and Science in Sports and Exercise, 50(3), 551–561. https://doi.org/10.1249/MSS.0000000000001451
Loucks, A. B., & Thuma, J. R. (2003). Luteinizing hormone pulsatility is disrupted at a threshold of energy availability in regularly menstruating women. Journal of Clinical Endocrinology and Metabolism, 88(1), 297–311. https://doi.org/10.1210/jc.2002-020369
Lundy, B., Torstveit, M. K., Stenqvist, T. B., Burke, L. M., Garthe, I., Slater, G. J., Ritz, C., & Melin, A. K. (2022). Screening for Low Energy Availability in Male Athletes: Attempted Validation of LEAM-Q. Nutrients, 14(9), 1873. https://doi.org/10.3390/nu14091873
Melin, A. K., Heikura, I. A., Tenforde, A., & Mountjoy, M. (2019). Energy availability in athletics: Health, performance, and physique. International Journal of Sport Nutrition and Exercise Metabolism, 29(2), 152–164. https://doi.org/10.1123/ijsnem.2018-0201
Mountjoy, M., Sundgot-Borgen, J., Burke, L., Carter, S., Constantini, N., Lebrun, C., Meyer, N., Sherman, R., Steffen, K., Budgett, R., & Ljungqvist, A. (2014). The IOC consensus statement: Beyond the Female Athlete Triad—Relative Energy Deficiency in Sport (RED-S). Sport En Geneeskunde, 47(4), 15–28. https://doi.org/10.1136/bjsports-2014-093502
Nattiv, A., de Souza, M. J., Koltun, K. J., Misra, M., Kussman, A., Williams, N. I., Barrack, M. T., Kraus, E., Joy, E., & Fredericson, M. (2021). The Male Athlete Triad—A Consensus Statement From the Female and Male Athlete Triad Coalition Part 1. Clinical Journal of Sport Medicine, Publish Ah(June). https://doi.org/10.1097/jsm.0000000000000946
Papageorgiou, M., Elliott-Sale, K. J., Parsons, A., Tang, J. C. Y., Greeves, J. P., Fraser, W. D., & Sale, C. (2017). Effects of reduced energy availability on bone metabolism in women and men. Bone, 105, 191–199. https://doi.org/10.1016/j.bone.2017.08.019
Reed, J. L., de Souza, J. J., Mallinson, R. J., Scheid, J. L., & Williams, N. I. (2015). Energy availability discriminates clinical menstrual status in exercising women. Journal of the International Society of Sports Nutrition, 12(1), 1–11. https://doi.org/10.1186/s12970-015-0072-0
Strock, N. C. A., Koltun, K. J., Southmayd, E. A., Williams, N. I., & de Souza, M. J. (2020). Indices of resting metabolic rate accurately reflect energy deficiency in exercising women. International Journal of Sport Nutrition and Exercise Metabolism, 30(1), 14–24. https://doi.org/10.1123/ijsnem.2019-0199
Tenforde, A. S., Barrack, M. T., Nattiv, A., & Fredericson, M. (2016). Parallels with the Female Athlete Triad in Male Athletes. Sports Medicine, 46(2), 171–182. https://doi.org/10.1007/s40279-015-0411-y
Yeager, K., Agostini, R., Nattive, A., & Drinkwater, B. (1993). The female athletic triad: Disordered eating, amenorrhea, and osteoporosis. In The Active Female: Health Issues Throughout the Lifespan, Second Edition (pp. 177–190). https://doi.org/10.1007/978-1-4614-8884-2_12