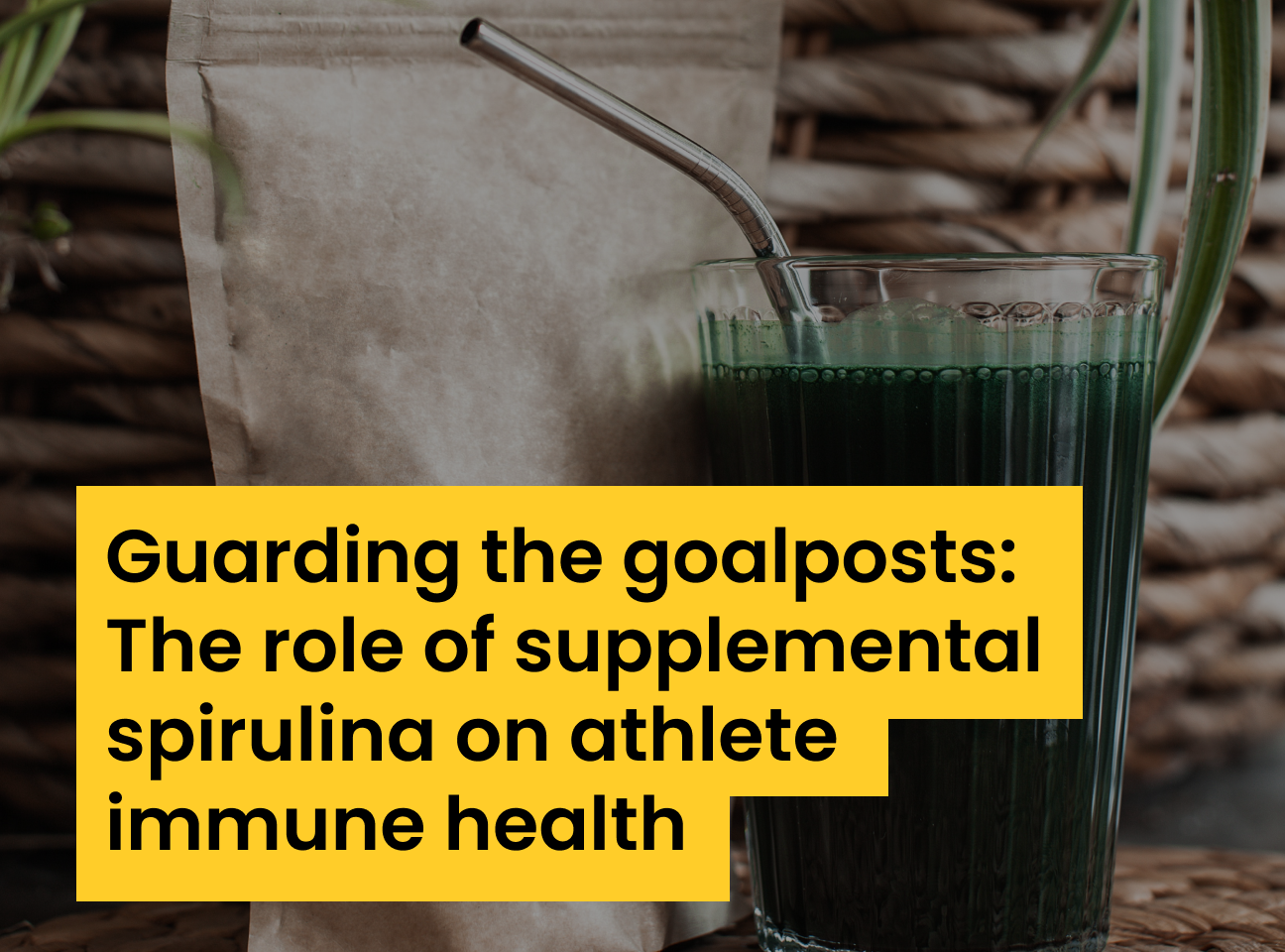
Guarding the goalposts: The role of supplemental spirulina on athlete immune health
By Alex Ritson MSc, Head of Education
Research: Effects on Spirulina Supplementation on Immune Cells’ Parameters of Elite College Athletes by Zhang et al. (2022)
Context:
Picture this: You’re an athlete, days away from one of the most important moments of your life — your event at the Olympics. The years of graft, the setbacks, the family and relationship events you’ve missed, all for this moment on the world’s biggest stage. Following several days of travel and on the heels of a rigorous training block, you arrive at the Olympic Village. You’re injury-free and pegged as a medal contender. But something isn’t quite right — your throat feels sore, and you struggle to breathe through your nose. You begin ruminating over what this could mean if symptoms worsen. The following day, the worst happens: you wake up with a low-grade fever, blocked nose, headache and a general feeling of malaise. You’ve contracted an upper respiratory tract infection (URTI) on the precipice of the most important day of your life. In the following days, you scramble with your athlete-support team, seeing what medications can be taken that adhere to WADA’s anti-doping rules and any immune-tolerating nutrients to hasten recovery. Perhaps symptoms will subside enough to compete, but with such fine margins between competitors, this infection has left you at a disadvantage. Alternatively, the worst occurs, you withdraw — a safety measure for your health, as strenuous exercise when fighting an infection can lead to myocarditis or rhabdomyolysis.
We’ve all experienced the annoyance of a URTI, but for athletes, a ‘cold’ can mean the difference between, quite literally, success or failure. While getting sick before competition presents an obvious example of how illness can impair an athlete’s performance, less obvious is the fact that illness, even during training, can reduce an athlete’s chances of success. For many athletes, training availability is an essential aspect of performance success (Raysmith & Drew, 2016). An illness-induced reduction in training volume can result in losses in training-induced adaptations, causing a reduction in performance. This is supported empirically, with medal winners suffering fewer and shorter infections (Hellard et al., 2015; Svendsen et al., 2016). While immunosuppression in athletes caused by exercise alone remains a hotly contested topic, being an athlete does lend itself to several immune challenges: contact with other athletes, support personnel and crowds (possibly infected); disrupted sleep, travel, insufficient energy and nutrient intake, and psychological stress. Irregular changes in the ratio of immune cells such as blood leukocytes, including granulocytes (neutrophils, eosinophils, and basophils), monocytes, and lymphocytes, commonly serve as proxies of immune health both in research and practice. This study aimed to assess whether spirulina, an extract of blue-green algae rich in tocopherols, β-carotene, polyphenols and phytocyanins, could serve as a supplement to support immune function in athletes enduring a strenuous eight-week training protocol.
The study:
Thirty-nine undergraduate male soccer players were randomly assigned to receive a daily dose of the treatment (3 g of spirulina supplementation; n=20) or placebo (n=19) throughout a high-intensity (~150 mins per day, six days per week) eight-week training protocol. Venous blood samples of leukocytes, specifically, granulocytes (neutrophils, eosinophils and basophils) and agranulocytes (monocytes and lymphocytes), were taken 24 hours before the first training session and 24 hours following the final training session after the eight-week protocol.
Essential knowledge: What are leukocytes?
Leukocytes is derived from the Greek word “leukos”, meaning white, and “kytos”, meaning cell. Ergo, leukocytes are the white blood cells (WBCs) of the body, residing at roughly 1:1000 red blood cells — this varies considerably depending on the health status of an individual. Like all blood cells, they originate from hematopoietic stem cells — “haima” and “poiein” in Greek, meaning “blood, and “to make” and occur predominantly in bone marrow. While we can think of red blood cells as key to athletic performance due to their role in oxygen transport, we can think of WBCs as key in our ability to fight against pathogens (biological agents that cause disease or illness). WBCs serve as the cellular components of the immune system. Notably, we also have soluble (e.g., inflammatory proteins) and physical barriers (e.g., skin and mucus) to our immune system. Unlike red blood cells, WBCs comprise diverse cell types that differ in abundance and function. Around 60-70% of WBCs are made of granulocytes, being neutrophils, eosinophils, and basophils. They are called ‘granulocytes’ because each of these WBCs contains granules (essentially small vesicles) containing various enzymes, proteins and other substances, which determine the function of the cell. For instance, neutrophils have specific enzymes, such as defensin, that kill and digest microorganisms; this process is called phagocytosis. I like to think of this process as a trash collector (neutrophil or macrophage) with a large garbage bag and utility belt lined with various disinfectant products. As the trash collector finds rubbish (pathogens), they are put inside a bag (engulfing the pathogen inside a vacuole). However, rather than just sealing the bag, they add several sprays of disinfectant (enzymes). This disinfectant neutralises the rubbish. Finally, the trash collector takes this waste to a disposal area (the phagolysosome), where it is destroyed. The granules within eosinophils serve as important combatants against large pathogens (parasites), with elevations in eosinophils typically seen in individuals with specific allergies (e.g., allergic rhinitis). Eosinophils also have antigen-presenting cell (APC) properties, which are like quality inspectors if we refer back to the trash-collecting analogy. Essentially, quality inspectors receive a sample of the trash (pathogen) and bring it to a meeting with environmental specialists (lymphocytes) to discern how to best deal with this type of waste, creating new processing methods to deal with similar waste products in the future (the adaptive immune system). Basophils are the least abundant WBCs. When activated, they release histamine from their granules, resulting in increased dilation and permeability of nearby blood capillaries; this allows for increased blood flow and the migration of other immune cells and proteins to the infected area. Basophils can be considered response coordinators in the analogy, whereby they assist in quickly mobilising trash collectors and other helpers to get rid of the rubbish. Monocytes and lymphocytes are referred to as ‘agranulocytes’, meaning they contain no granules in their cytoplasm. Monocytes are the largest WBCs and can differentiate into macrophages and dendritic cells; essentially, they’re like utility workers with the capacity to perform many functions. The four essential functions they perform are phagocytosis (waste disposal), intracellular killing (another form of waste disposal), antigen presentation (quality assessment), and cytokine production. The latter function can be thought of as walkie-talkies in the trash collecting operation, signalling where waste can be found. Lymphocytes can be broadly categorised into T and B cells, which are involved in cell-mediated and humoral immunity, respectively. We can think of cell-mediated as door-to-door trash collecting and humoral immunity as a system that tags specific trash for collection and neutralisation.
A closer look: The biphasic response of leukocytes to exercise: Challenging the ‘open window’ hypothesis
Exercise causes a biphasic (two distinct phases) response in blood leukocyte concentrations during and post-exercise. During training, circulatory leukocyte levels increase, called ‘lymphocytosis’, due to adrenergic stimulation and fall below pre-exercise baseline levels for 1-2 hours following exercise, called ‘lymphopenia’. This biphasic response is also why the researchers decided to wait 24 hours before and after training to assess blood leukocyte levels. Previous reports of lymphopenia in the initial hours following intense exercise suggested that strenuous exercise suppresses the immune system and increases an athlete’s susceptibility to opportunistic infection. This idea was termed the ‘open window’ hypothesis. However, recent evidence suggests that transient lymphopenia reflects a redistribution of leukocytes to peripheral tissues (e.g., the gut, lungs, and bone marrow). It illustrates a heightened state of immune surveillance against potential pathogens. Thus, this transient decrease in leukocyte levels may not be a sign of immunosuppression but a sign of a healthy, well-functioning immune system (Campbell & Turner, 2018).
Findings:
Following the end of the eight weeks of high-intensity training, blood measures from the placebo group demonstrated statistically significant falls in total leukocyte count (p <0.04) and monocytes (p <0.01). In contrast, changes in the spirulina group remained insignificant, apart from eosinophil, which increased. Moreover, those in the spirulina group demonstrated a higher average of both monocytes (p <0.05) and basophils (p <0.05) compared to the placebo group.
Relevance:
The study found that 3g per day of spirulina supplementation following 8 weeks of intense exercise helped prevent reductions in leukocytes, eosinophils, basophils, and monocytes. However, no changes were seen in neutrophils or lymphocytes. This suggests that spirulina may impact only specific immune cells. Despite statistically significant reductions in the placebo group, immune cell concentrations remained within the normal clinical reference ranges, suggesting that 8 weeks of intense exercise may cause changes in specific immune cells but does not indicate clinical immune suppression. Moreover, it’s key to understand that blood measures of immune cell count only serve as proxies of immune health; they do not provide insight into the function or activation of immune cells. As an analogy, it’s like counting the number of players on a soccer team. This does not provide any indication of the skill level of the group. To discern the team’s quality, you need to assess them in action: their technique, speed, and intelligence. This is akin to performing immune measurements, such as flow cytometry, salivary IgA, and vaccination responses, which provide a more meaningful picture of immune function than immune cell count.
Moreover, one of spirulina’s functional roles is acting as a free-radical scavenger. It’s important to note that free-radical production following exercise is an essential modulator of training adaptation. Therefore, hypothetically, chronic consumption of spirulina, particularly if consumed post-exercise, might dampen the adaptive response from training, although this is yet to be empirically verified, and thus, the dosage and timing of spirulina supplementation require consideration by the practitioner.
Practical translation:
These results suggest that spirulina supplementation may benefit certain immune cells following intense exercise. Considering the methodology used and the potential negative training adaptation implications, using spirulina supplementation for immune support, akin to the bulk of “immune-boosting” supplements, remains questionable.
Recommended reading:
Nutrition and Athlete Immune Health: New Perspectives on an Old Paradigm by Walsh et al. (2019) (open access)
Complement this research with the We Do Science podcast episode:
Exercise, Immune Function and Infection Risk with Dr Richard Simpson
References:
Campbell, J. P., & Turner, J. E. (2018). Debunking the Myth of Exercise-Induced Immune Suppression: Redefining the Impact of Exercise on Immunological Health Across the Lifespan. Frontiers in Immunology, 9, 648. https://doi.org/10.3389/fimmu.2018.00648
Hellard, P., Avalos, M., Guimaraes, F., Toussaint, J.-F., & Pyne, D. B. (2015). Training-Related Risk of Common Illnesses in Elite Swimmers over a 4-yr Period. Medicine & Science in Sports & Exercise, 47(4), 698. https://doi.org/10.1249/MSS.0000000000000461
Raysmith, B. P., & Drew, M. K. (2016). Performance success or failure is influenced by weeks lost to injury and illness in elite Australian track and field athletes: A 5-year prospective study. Journal of Science and Medicine in Sport, 19(10), 778–783. https://doi.org/10.1016/j.jsams.2015.12.515
Svendsen, I. S., Taylor, I. M., Tønnessen, E., Bahr, R., & Gleeson, M. (2016). Training-related and competition-related risk factors for respiratory tract and gastrointestinal infections in elite cross-country skiers. British Journal of Sports Medicine, 50(13), 809–815. https://doi.org/10.1136/bjsports-2015-095398