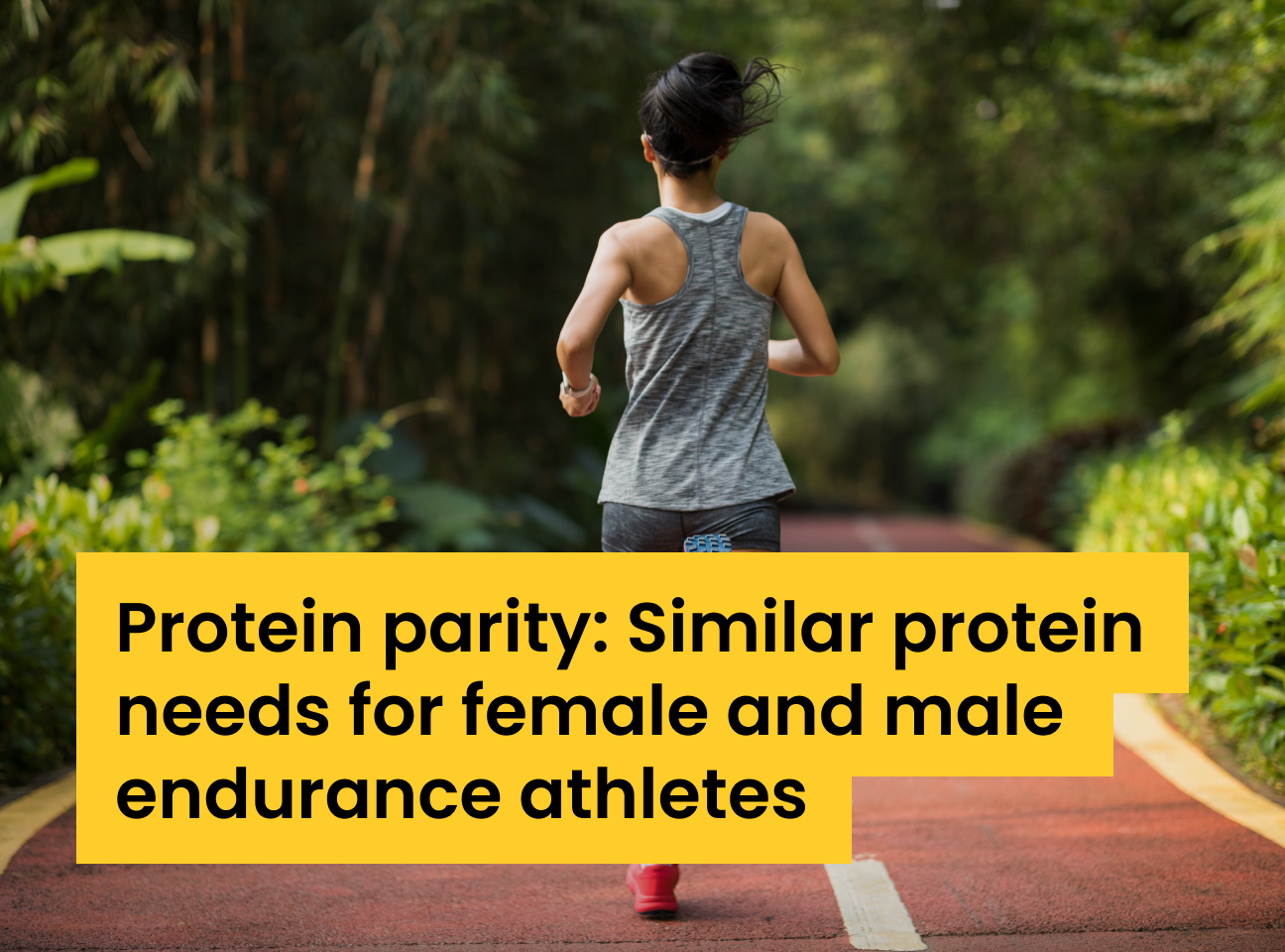
Protein parity: Similar protein needs for female and male endurance athletes
By Alex Ritson MSc, Head of Education
Research: Protein Requirements Are Increased in Endurance-Trained Athletes but Similar between Females and Males during Postexercise Recovery by Williamson et al. (2023)
Introduction
Building muscle and lifting weights are usually top-of-mind when thinking of dietary protein and exercise, not its role in supporting endurance performance. But perhaps it’s time our cognitive settings got an ‘update’ on this perspective. Recent research suggests that the protein requirements for resistance and endurance athletes are similar (~1.6-1.8 g protein.kg⁻¹.d⁻¹) (Kato et al., 2016; Morton et al., 2018). Our cognitive bias towards protein and muscle-building is partly due to science. The connection and underlying mechanisms for dietary protein paired with resistance exercise on synthesising myofibrillar proteins (the contractile units of the muscle) and increasing skeletal muscle size have been widely studied and well-established (Stokes et al., 2018). Comparatively, less research has explored the role of protein in supporting endurance performance and adaptation. But a more precise picture is starting to emerge. What we do know is that elevated protein requirements for endurance athletes are partly due to the increased oxidation of amino acids during exercise (Tarnopolsky, 2004). What’s more, endurance exercise increases the synthesis and remodelling of mitochondrial proteins, thereby enhancing muscle oxidative capacity (Churchward-Venne et al., 2020; Wilkinson et al., 2008). In addition, it’s been theorised that the elevated protein requirements for the endurance athlete relate to the increased need for enzymes, for capillarisation, and haemoglobin and myoglobin synthesis (Knuiman et al., 2018; Tarnopolsky, 2004). An overview of the potential roles of protein as it relates to endurance-training-induced adaptations is shown in Figure 1. However, the research for determining protein recommendations in endurance athletes — much like most research in sport and exercise nutrition — has been established only in male participants. Therefore, questions remain on whether these recommendations can be applied to females. To address this, this research aimed to determine the protein requirements of male and female athletes outside of the laboratory (home-based setting) using the indicator amino acid oxidation (IAAO) method. The group hypothesised that:
- The oxidation rate of the indicator amino acid (phenylalanine) would be lowest at the highest protein intake.
- The breakpoint analysis (recommended intake) would be similar to previous laboratory research (e.g., 1.83 g protein.kg⁻¹.d⁻¹) (Kato et al., 2016).
- The breakpoint (e.g., protein requirements) in female participants would be lower than similarly trained males.
Figure 1. A simplified overview representing the regulation of endurance trained-induced adaptations and the potential role of protein. Retrieved from Knuiman et al. (2018)
A closer look: What is the IAAO method for measuring whole-body protein synthesis?
The IAAO method is a technique for indirectly measuring protein synthesis rates and thus determining protein requirements. The method is based on measuring the oxidation rate of an indicator essential amino acid (EAA) when another EAA is deficient. Put simply, the method assumes that if one EAA is deficient for protein synthesis, then the excess of all other EAA will be oxidised, including the indicator amino acid. Let me explain using the analogy of building a house. Think of protein synthesis like the process of building a home. The EAAs are like the critical building materials: cement, bricks, wooden beams, etc, and the building crew are like the essential enzymes and regulatory molecules that facilitate the building process. Imagine that at the building site, all the crew are ready to work. What’s more, all the essential materials are on location except one: cement. Of course, the crew can make some progress by putting up scaffolding, laying down a few bricks and wooden beams, but proper house building can’t occur without cement. Due to there being no safe storage area on site (amino acids cannot be stored for later use), the excess building materials are sent back to the quarry (akin to the excess EAAs being oxidised). This analogy underscores a fundamental principle of protein synthesis: all EAAs are required for effective protein synthesis (e.g., house building). The IAAO method uses this principle to determine protein synthesis rates indirectly. As amino acids cannot be stored for later use, EAAs have two metabolic fates: synthesis or oxidation. The EAA phenylalanine is typically used as the indicator amino acid, whereby its metabolic fate is ‘traced’ via participants consuming a stable isotope labelled form of phenylalanine (L-[1-¹³CO] phenylalanine). Measuring changes in phenylalanine excretion/production (e.g., phenylalanine oxidation) in urine and breath samples from participants indirectly estimates the incorporation of phenylalanine into protein (e.g., protein synthesis). Consuming higher amounts of protein will result in a corresponding decrease in phenylalanine oxidation and, thus, an indication of increased whole body protein synthesis rates. The breakpoint defines a plateau in phenylalanine oxidation rates and indicates the maximal rate of protein synthesis and subsequent protein requirements for the participant.
The study:
This was a randomised, counterbalanced, within-participant design. Eight male (~30 yr) and seven female (~30 yr) trained endurance runners (≥5h of running per week with a “superior” VO₂max as categorised by ACSM) were included in the analysis. All female participants undertook the experiment during the luteal phase of their cycle. The study was carried out during the COVID-19 pandemic, which meant the experiments took place at each participant’s home. Each participant adhered to three trial periods consisting of three days each. For the first two days, they followed a controlled diet (1.4 g protein.kg⁻¹.d⁻¹) and adhered to 10 km (day 1) and 5 km (day 2) running sessions, respectively. On day three, runners completed a 20 km run before starting the metabolic trial using a modified version of the IAAO technique. Following the run, researchers instructed participants via video to consume the test drinks each hour, some of which were enriched with the stable isotope tracer L-[¹³C] phenylalanine. The participants collected their own breath and urine samples before being retrieved by researchers. The three protein intakes during the three different trial periods were as follows:
- A suboptimal protein intake (0.2 g. kg⁻¹. d⁻¹) for characterising the first ‘slope’ in the breakpoint analysis — a point which underscores a change in phenylalanine oxidation or whole body protein synthesis due to insufficient protein intake.
- A moderate protein intake (1.2 g. kg⁻¹. d⁻¹) per the lower range of the ACSM protein recommendations for athletes.
- A higher protein intake (2.0 g. kg⁻¹. d⁻¹), per the upper boundary of protein requirements using the IAAO method in male endurance athletes (Kato et al., 2016).
Findings:
To recap, this research aimed to determine the protein needs of male and female endurance athletes using a modified three-point (three different protein intakes) version of the IAAO method in a free-living context. The three levels of protein intake prescribed (0.2, 1.2, 2.0 g.kg⁻¹.d⁻¹) had a significant impact on phenylalanine oxidation rates, with higher protein levels causing a stepwise reduction in phenylalanine oxidation but with no sex-based difference. The breakpoint (the threshold where phenylalanine oxidation plateaued) was virtually identical between both sexes: 1.60 g.kg⁻¹.d⁻¹ for males and 1.61 g.kg⁻¹.d⁻¹ for females, respectively. Using the upper 95% confidence interval, the researchers determined that protein requirements for endurance athletes should be 1.81 and 1.89 g.kg⁻¹.d⁻¹ for male and female athletes, respectively.
Relevance:
Due to COVID restrictions, this research group implemented the first study to prospectively determine the protein needs of endurance athletes in a free-living setting. They achieved this by using an adapted three-point IAAO technique. While performing this technique outside of the controlled confines of a laboratory increases the susceptibility for bias, the method resulted in almost identical results to the traditional IAAO method in a laboratory setting. This study demonstrated that endurance athletes performing a 20 km run require a protein intake of 1.81 and 1.89 g.kg⁻¹.d⁻¹ for male and female athletes, respectively. For simplicity, the researchers suggest a general protein recommendation of 1.85 g.kg⁻¹.d⁻¹ to maximise whole-body protein synthesis for endurance athletes (independent of sex) or 0.46 g.kg of protein per meal over four meals. Notably, this is ~2.3 times greater than the current RDA (based on nitrogen balance) protein recommendations for healthy adults (0.8 g.kg⁻¹.d⁻¹) and 55% greater than recommendations for non-exercising individuals (based on the IAAO technique). While the mechanisms for why the protein requirements were elevated in endurance athletes were not studied, the elevation was likely due, in part, to the roles outlined in Figure 1. However, something the reader should bear in mind is that protein requirements were determined via the consumption of a crystalline amino acid mixture, not whole foods. However, whether this will result in significant changes in whole-body protein synthesis compared to consuming whole-food sources of protein requires further study, but recent evidence suggests there is minimal difference (Weijzen et al., 2022).
Complement this research with the We Do Science podcast episode: Protein and Endurance Athletes with Dan Moore PhD
References:
Churchward-Venne, T. A., Pinckaers, P. J., Smeets, J. S., Betz, M. W., Senden, J. M., Goessens, J. P., Gijsen, A. P., Rollo, I., Verdijk, L. B., & Van Loon, L. J. (2020). Dose-response effects of dietary protein on muscle protein synthesis during recovery from endurance exercise in young men: A double-blind randomized trial. The American Journal of Clinical Nutrition, 112(2), 303–317. https://doi.org/10.1093/ajcn/nqaa073
Kato, H., Suzuki, K., Bannai, M., & Moore, D. R. (2016). Protein Requirements Are Elevated in Endurance Athletes after Exercise as Determined by the Indicator Amino Acid Oxidation Method. PloS One, 11(6), e0157406. https://doi.org/10.1371/journal.pone.0157406
Knuiman, P., Hopman, M. T. E., Verbruggen, C., & Mensink, M. (2018). Protein and the Adaptive Response With Endurance Training: Wishful Thinking or a Competitive Edge? Frontiers in Physiology, 9, 598. https://doi.org/10.3389/fphys.2018.00598
Morton, R. W., Murphy, K. T., McKellar, S. R., Schoenfeld, B. J., Henselmans, M., Helms, E., Aragon, A. A., Devries, M. C., Banfield, L., Krieger, J. W., & Phillips, S. M. (2018). A systematic review, meta-analysis and meta-regression of the effect of protein supplementation on resistance training-induced gains in muscle mass and strength in healthy adults. British Journal of Sports Medicine, 52(6), 376–384. https://doi.org/10.1136/bjsports-2017-097608
Stokes, T., Hector, A. J., Morton, R. W., McGlory, C., & Phillips, S. M. (2018). Recent Perspectives Regarding the Role of Dietary Protein for the Promotion of Muscle Hypertrophy with Resistance Exercise Training. Nutrients, 10(2), Article 2. https://doi.org/10.3390/nu10020180
Tarnopolsky, M. (2004). Protein requirements for endurance athletes. Nutrition, 20(7), 662–668. https://doi.org/10.1016/j.nut.2004.04.008
Weijzen, M. E. G., van Gassel, R. J. J., Kouw, I. W. K., Trommelen, J., Gorissen, S. H. M., van Kranenburg, J., Goessens, J. P. B., van de Poll, M. C. G., Verdijk, L. B., & van Loon, L. J. C. (2022). Ingestion of Free Amino Acids Compared with an Equivalent Amount of Intact Protein Results in More Rapid Amino Acid Absorption and Greater Postprandial Plasma Amino Acid Availability Without Affecting Muscle Protein Synthesis Rates in Young Adults in a Double-Blind Randomized Trial. The Journal of Nutrition, 152(1), 59–67. https://doi.org/10.1093/jn/nxab305
Wilkinson, S. B., Phillips, S. M., Atherton, P. J., Patel, R., Yarasheski, K. E., Tarnopolsky, M. A., & Rennie, M. J. (2008). Differential effects of resistance and endurance exercise in the fed state on signalling molecule phosphorylation and protein synthesis in human muscle. The Journal of Physiology, 586(Pt 15), 3701–3717. https://doi.org/10.1113/jphysiol.2008.153916