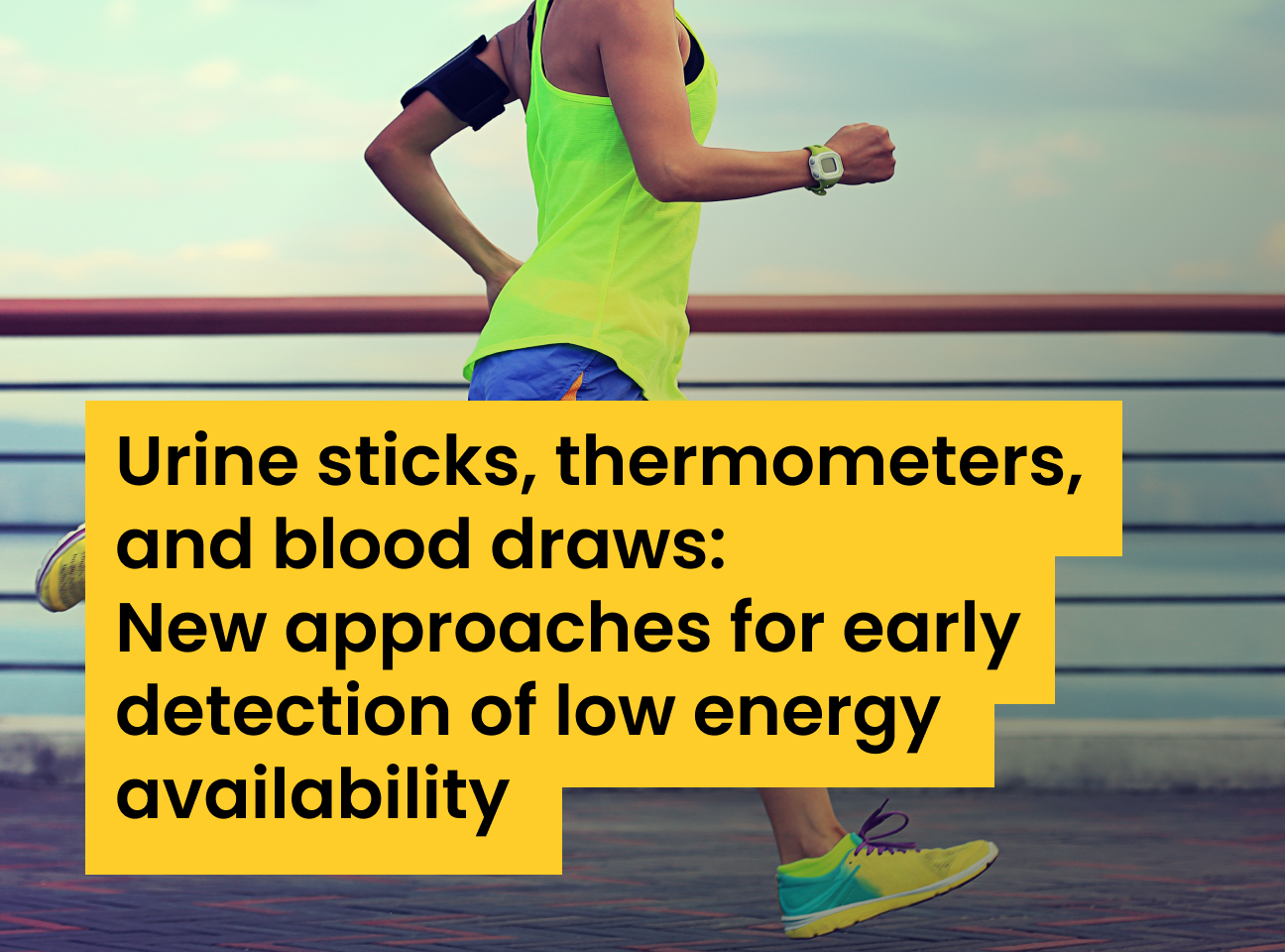
Urine sticks, thermometers, and blood draws: New approaches for early detection of low energy availability
By Alex Ritson MSc, Head of Education
Research: Free-living competitive racewalkers and runners with energy availability estimates of <35 kcal·kg fat-free mass−1·day−1 exhibit peak serum progesterone concentrations indicative of ovulatory disturbances: a pilot study by Castellanos-Mendoza, Galloway & Witard (2023) (open access)
Introduction
Like a company managing the ebbs and flows of its budget, prioritising essential expenses (think employee wages) before discretionary spending (think marketing and advertising); humans (and all other mammals) are continually balancing their own set of processes. However, in our case, our problem isn’t money (well, not physiologically); it’s energy. Contrary to what our overstuffed supermarket aisles imply, we (Homo sapiens) are far more attuned to living/surviving in a state of food scarcity, not surplus. It’s just the last ~200 years (0.007%) of our ~300,000-year existence where some of us have lived with surplus food. Over 299,800 years, we developed mechanisms to combat food scarcity by allocating our finite energy reserves to body systems that determine our immediate survival (e.g., neural activity and cardiac output) while temporarily down-regulating other biological processes (e.g., growth and reproduction). This tactful delegation of energy reserves is called energy availability (EA).
In sport and exercise nutrition, EA refers to left over energy (kilocalories; kCal) reserves to support physiological processes after accounting for the difference between dietary energy intake (kCal) and exercise energy expenditure (kCal) (Loucks et al., 2011). The negative health effects of low EA have been estimated to occur at <30 kCal/kg FFM-1.day−1 in sedentary women in laboratory conditions, whereby luteinising hormone (LH) pulsatility reduces — a proxy for reproductive activity (Loucks & Thuma, 2003). Moreover, a healthy EA is estimated to occur at ~45 kCal/kg FFM-1.day−1 (Loucks et al., 2011). Female and male athletes competing in sports that emphasise leanness or with high energy expenditure rates — whether deliberately, compulsively, or unintentionally — commonly demonstrate signs of low EA. In a state of low EA, energy is repartitioned away from reproductive function, along with other processes, such as muscle protein and glycogen synthesis and bone metabolism, impairing exercise capacity, recovery, and increasing the risk of injury (Mountjoy et al., 2023).
While the impact of low EA can independently disrupt bone metabolism, the suppression of reproductive hormones (e.g., oestrogen) may have combined and independent negative effects on bone (Southmayd et al., 2017), further increasing an athlete’s risk to bone-stress injuries. The relationship between EA, menstrual function and bone metabolism is well established and was initially defined as the Female Athlete Triad (Yeager et al., 1993), evolving to include male athletes (Nattiv et al., 2021). The Triad also makes up a part of a larger concept, known as Relative Energy Deficiency in Sport (RED-S) (Mountjoy et al., 2014, 2018, 2023), which underscores a series of interconnected health and performance decrements associated with low EA. Direct measures of EA require the practitioner to collect dietary energy intake (kCal) and exercise energy expenditure (kCal) data from an athlete, as well as a measure of fat-free mass (FFM). However, current field measures for dietary energy intake, exercise energy expenditure and body composition remain inaccurate and unreliable, limiting the effectiveness of measuring EA outside of laboratory conditions (Burke et al., 2018). Instead, a recent review has suggested adopting a triangulated approach, using estimates of EA alongside other indirect measures of energy status (e.g., resting metabolic rate, anthropometric characteristics, and metabolic/hormonal status) (De Souza et al., 2019). As reproductive function is one of the first physiological systems to constrain during a state of low EA (Wade & Jones, 2004), changes in reproductive hormones during specific phases of a menstrual cycle could serve as early proxy signs of energy deficiency.
The menstrual cycle is a monthly, periodic process that prepares a woman for pregnancy. It consists of four stages: menstruation, the follicular phase, ovulation, and the luteal phase (Elliott-Sale et al., 2021). During different stages of the cycle, the interplay of various hormones, namely oestrogen and progesterone — which fluctuate 5 and 50-fold over a menstrual cycle (Elliott-Sale et al., 2021) — cause various physiological changes. One notable change is the slight rise in basal body temperature, commonly used as an indicator of ovulation.
This proof-of-concept study explored the relationship between field measures of EA and ovulatory status (e.g., menstrual cycle function) via serum peak progesterone concentrations, alongside measures of LH and basal body temperature, in regularly menstruating endurance athletes.
The study:
This was a prospective observational pilot study. Akin to a TV pilot, it serves as a “test run” to gauge its potential for success. “Success” for the TV pilot refers to audience engagement and interest, whereas for a research study, it relates to its feasibility and validity. Specifically, whether the research methods are accurate and reliable and if the investigation can be performed within the constraints of time, funding, and resources.
The study observed trained (tier 2) and elite/international (tier 4) female racewalkers (n=8) and runners (n=7), as categorised by McKay et al. (2022), in a free-living environment. Only participants deemed “not at risk of low energy availability” (score <8 points) per the LEAF questionnaire and who maintained a regular menstrual cycle for three consecutive months before the study were included in the investigation.
Each athlete’s EA was measured on days 3-12 of their menstrual cycle via weighed food and remote-food photography methods (kCal intake), energy expenditure data using metabolic equivalents of task (kCal expenditure), and skinfold thickness assessments (body fat predicted estimate of fat-free mass). Reproductive status was determined using LH test kits (via urine), blood draws for progesterone, and basal body temperature to verify each athlete’s menstrual cycle phase, as shown in Figure 1.
LH peak was determined with a reading of ≥30 mIU.ml−1, with ovulation assumed to occur the day after. Six to nine days following LH peak, serum measures of progesterone were taken from each athlete. Ovulatory status was categorised based on progesterone levels: anovulatory (≤6.00 ng·mL−1), luteal phase defect (6.01–9.40 ng.mL−1), and potential fertile or “normal ovulatory” status (>9.40 ng·mL−1). Body temperature was used to determine the menstrual phase, with three consecutive basal body temperature readings higher than the previous six days used to indicate ovulation.
Figure 1. An overview of the study protocol during a 28-day menstrual cycle. Retrieved from Castellanos-Mendoza et al. (2023)
Findings:
To recap, the study aimed to determine the relationship between measured EA and ovulatory status via peak progesterone concentrations in free-living, regularly menstruating athletes. The researchers found athletes with an EA of <35 kCal.kg.FFM-1.day−1 all showed signs of menstrual disturbance (anovulation, short luteal phase, and luteal-phase deficiency). Whereas athletes with an EA ≥36 kcal.kg FFM−1.day−1 had progesterone levels indicative of regular ovulation, except one athlete who reported the lowest protein and highest refined sugar intake. The researchers performed a regression analysis and found a positive correlation (r=0.79) between EA and serum progesterone concentrations once athletes suspected of under-reporting their dietary intake were omitted. Thus, using their field-based methods for tracking the menstrual cycle and EA, they found a positive relationship between reduced EA and ovulatory disturbance.
Relevance:
This investigation, using field-based measures of EA and urine (LH peak) and serum (progesterone peak) measurements to determine ovulatory status, showed a moderate, positive correlation (r=0.79) for athletes who reported an EA of <35 kcal.kg FFM−1.day−1 with ovulatory disturbance (peak/mid-luteal progesterone ≤9.40 ng.mL−1). Moreover, normal ovulatory status was associated with a higher EA of ≥36 kcal.kg FFM−1.day−1. These findings indicate that using basal body temperature with LH peak via urine samples and serum measures of peak/mid-luteal progesterone concentrations can detect an ovulatory disturbance in female athletes. Moreover, these disturbances were aligned with practical-field-based measures of low EA.
The measures adopted in this pilot study present a method for monitoring menstrual cycle status in athletes without needing to wait for signs of clinical menstrual dysfunction (absence of menstruation). With suppressed reproductive hormones and menstrual disturbance because of low EA associated with suppressed bone metabolism (Ihle & Loucks, 2004; Southmayd et al., 2017), resting metabolism (De Souza et al., 2007), and impaired athletic performance (VanHeest et al., 2014), this approach may provide a way to detect female athletes with low EA early before physiological suppression causes serious harm to the athlete (e.g., injury).
Notably, the results suggest that field measures of EA <35 kcal.kg FFM−1.day−1 may indicate ovulatory disturbance in females, thus a potential threshold for low EA. However, practitioners are advised to treat this index (<35 kcal·kg FFM−1 ·day−1) not as a binary threshold but rather as a potential indicator of low EA — due to the errors associated with collecting EA data in free-living conditions. Instead, practitioners can use this index (if adopting the same measurement practices) as a potential sign of low EA in female athletes. Furthermore, the practitioner should verify the athlete’s EA status by comparing this index with other indirect measures of energy deficiency, such as ovulatory status, as illustrated in this study.
Take home: For female athletes susceptible to low EA, during peak phases of their training/competition, practitioners may want to consider monitoring their athlete’s ovulatory status (adopting the methods shown in Figure 1). Doing so will ensure they can proactively identify and address signs of energy deficiency early, thereby preventing severe low EA-related performance or health issues for the athlete.
Complement this research by reading this article: 2023 International Olympic Committee’s (IOC) consensus statement on Relative Energy Deficiency in Sport (REDs) by Mountjoy et al. (2023)
References:
Burke, L. M., Lundy, B., Fahrenholtz, I. L., & Melin, A. K. (2018). Pitfalls of Conducting and Interpreting Estimates of Energy Availability in Free-Living Athletes. International Journal of Sport Nutrition and Exercise Metabolism, 28(4), 350–363. https://doi.org/10.1123/ijsnem.2018-0142
De Souza, M. J., Koltun, K. J., Strock, N. C., & Williams, N. I. (2019). Rethinking the concept of an energy availability threshold and its role in the Female Athlete Triad. Current Opinion in Physiology, 10, 35–42. https://doi.org/10.1016/j.cophys.2019.04.001
De Souza, M. J., Lee, D. K., VanHeest, J. L., Scheid, J. L., West, S. L., & Williams, N. I. (2007). Severity of energy-related menstrual disturbances increases in proportion to indices of energy conservation in exercising women. Fertility and Sterility, 88(4), 971–975. https://doi.org/10.1016/j.fertnstert.2006.11.171
Elliott-Sale, K. J., Minahan, C. L., de Jonge, X. A. K. J., Ackerman, K. E., Sipilä, S., Constantini, N. W., Lebrun, C. M., & Hackney, A. C. (2021). Methodological Considerations for Studies in Sport and Exercise Science with Women as Participants: A Working Guide for Standards of Practice for Research on Women. Sports Medicine (Auckland, N.z.), 51(5), 843–861. https://doi.org/10.1007/s40279-021-01435-8
Ihle, R., & Loucks, A. B. (2004). Dose-response relationships between energy availability and bone turnover in young exercising women. Journal of Bone and Mineral Research: The Official Journal of the American Society for Bone and Mineral Research, 19(8), 1231–1240. https://doi.org/10.1359/JBMR.040410
Loucks, A. B., Kiens, B., & Wright, H. H. (2011). Energy availability in athletes. Journal of Sports Sciences, 29(sup1), S7–S15. https://doi.org/10.1080/02640414.2011.588958
Loucks, A. B., & Thuma, J. R. (2003). Luteinizing hormone pulsatility is disrupted at a threshold of energy availability in regularly menstruating women. The Journal of Clinical Endocrinology and Metabolism, 88(1), 297–311. https://doi.org/10.1210/jc.2002-020369
Mountjoy, M., Ackerman, K. E., Bailey, D. M., Burke, L. M., Constantini, N., Hackney, A. C., Heikura, I. A., Melin, A., Pensgaard, A. M., Stellingwerff, T., Sundgot-Borgen, J. K., Torstveit, M. K., Jacobsen, A. U., Verhagen, E., Budgett, R., Engebretsen, L., & Erdener, U. (2023). 2023 International Olympic Committee’s (IOC) consensus statement on Relative Energy Deficiency in Sport (REDs). British Journal of Sports Medicine, 57(17), 1073–1097. https://doi.org/10.1136/bjsports-2023-106994
Mountjoy, M., Sundgot-Borgen, J., Burke, L., Carter, S., Constantini, N., Lebrun, C., Meyer, N., Sherman, R., Steffen, K., Budgett, R., & Ljungqvist, A. (2014). The IOC consensus statement: Beyond the Female Athlete Triad—Relative Energy Deficiency in Sport (RED-S). British Journal of Sports Medicine, 48(7), 491–497. https://doi.org/10.1136/bjsports-2014-093502
Mountjoy, M., Sundgot-Borgen, J. K., Burke, L. M., Ackerman, K. E., Blauwet, C., Constantini, N., Lebrun, C., Lundy, B., Melin, A. K., Meyer, N. L., Sherman, R. T., Tenforde, A. S., Torstveit, M. K., & Budgett, R. (2018). IOC consensus statement on relative energy deficiency in sport (RED-S): 2018 update. British Journal of Sports Medicine, 52(11), 687–697. https://doi.org/10.1136/bjsports-2018-099193
Nattiv, A., De Souza, M. J., Koltun, K. J., Misra, M., Kussman, A., Williams, N. I., Barrack, M. T., Kraus, E., Joy, E., & Fredericson, M. (2021). The Male Athlete Triad-A Consensus Statement From the Female and Male Athlete Triad Coalition Part 1: Definition and Scientific Basis. Clinical Journal of Sport Medicine: Official Journal of the Canadian Academy of Sport Medicine, 31(4), 335–348. https://doi.org/10.1097/JSM.0000000000000946
Southmayd, E. A., Mallinson, R. J., Williams, N. I., Mallinson, D. J., & De Souza, M. J. (2017). Unique effects of energy versus estrogen deficiency on multiple components of bone strength in exercising women. Osteoporosis International: A Journal Established as Result of Cooperation between the European Foundation for Osteoporosis and the National Osteoporosis Foundation of the USA, 28(4), 1365–1376. https://doi.org/10.1007/s00198-016-3887-x
VanHeest, J. L., Rodgers, C. D., Mahoney, C. E., & De Souza, M. J. (2014). Ovarian Suppression Impairs Sport Performance in Junior Elite Female Swimmers. Medicine & Science in Sports & Exercise, 46(1), 156. https://doi.org/10.1249/MSS.0b013e3182a32b72
Wade, G. N., & Jones, J. E. (2004). Neuroendocrinology of nutritional infertility. American Journal of Physiology-Regulatory, Integrative and Comparative Physiology, 287(6), R1277–R1296. https://doi.org/10.1152/ajpregu.00475.2004
Yeager, K. K., Agostini, R., Nattiv, A., & Drinkwater, B. (1993). The female athlete triad: Disordered eating, amenorrhea, osteoporosis. Medicine and Science in Sports and Exercise, 25(7), 775–777. https://doi.org/10.1249/00005768-199307000-00003